Next: Fine Structure of Hydrogen
Up: Relativistic Electron Theory
Previous: Electron Spin
To further study the motion of an electron in a central field, whose Hamiltonian is
it is convenient to transform to polar coordinates. Let
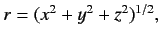 |
(1219) |
and
 |
(1220) |
It is easily demonstrated that
![$\displaystyle [r,p_r] = {\rm i} \,\hbar,$](img2784.png) |
(1221) |
which implies that in the Schrödinger representation
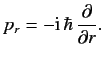 |
(1222) |
Now, by symmetry, an energy eigenstate in a central field is a simultaneous eigenstate of the total angular momentum
Furthermore, we know from general principles that the eigenvalues of
are
, where
is a positive half-integer (since
, where
is the standard non-negative integer quantum number associated with orbital angular momentum.)
It follows from Equation (1192) that
However, because
is an angular momentum, its components satisfy the standard commutation relations
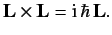 |
(1225) |
Thus, we obtain
However,
, so
Further application of (1192) yields
However, it is easily demonstrated from the fundamental commutation relations between position and momentum operators that
 |
(1230) |
Thus,
which implies that
Now,

. Moreover,
commutes with
,
, and
. Hence,
we conclude that
Finally, since
commutes with
and
, but anti-commutes with the components of
, we obtain
where
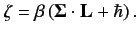 |
(1235) |
If we repeat the above analysis, starting at Equation (1228), but substituting
for
, and
making use of the easily demonstrated result
 |
(1236) |
we find that
Now,
commutes with
, as well as the components of
and
. Hence,
![$\displaystyle [\zeta,r] = 0.$](img2820.png) |
(1238) |
Moreover,
commutes with the components of
, and can easily be shown to commute with all components of
.
It follows that
![$\displaystyle [\zeta,\beta]=0.$](img2821.png) |
(1239) |
Hence, Equations (1218), (1234), (1238), and (1239) imply that
![$\displaystyle [\zeta, H] =0.$](img2822.png) |
(1240) |
In other words, an eigenstate of the Hamiltonian is a simultaneous eigenstate of
.
Now,
where use has been made of Equation (1227), as well as
. It follows that the eigenvalues of
are
. Thus, the eigenvalues of
can be written
, where
is a non-zero integer.
Equation (1192) implies that
where use has been made of (1220) and (1235).
It is helpful to define the new operator
, where
Moreover, it is evident that
![$\displaystyle [\epsilon,r] = 0.$](img2839.png) |
(1244) |
Hence,
where use has been made of (1117).
It follows that
 |
(1246) |
We have already seen that
commutes with
and
. Thus,
![$\displaystyle [\zeta,\epsilon] = 0.$](img2844.png) |
(1247) |
Equation (1192) gives
where use has been made of the fundamental commutation relations for position and momentum operators.
However,
and
, so, multiplying through by
, we get
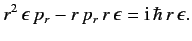 |
(1249) |
Equation (1221) then yields
![$\displaystyle [\epsilon,p_r]= 0.$](img2852.png) |
(1250) |
Equation (1242) implies that
Making use of Equations (1238), (1243), (1244), and (1246), we get
  |
(1252) |
Hence, the Hamiltonian (1218) becomes
 |
(1253) |
Now, we wish to solve the energy eigenvalue problem
 |
(1254) |
where
is the energy eigenvalue. However, we have already shown that an eigenstate of the Hamiltonian is a simultaneous eigenstate of the
operator belonging to the eigenvalue
, where
is a non-zero integer. Hence, the eigenvalue problem reduces to
![$\displaystyle \left[- e\,\phi(r) + c\,\epsilon\,(p_r-{\rm i}\,\hbar/r) + {\rm i}\,c\,\hbar\,k\,\epsilon\,\beta/r + \beta\,m_e\,c^2\right]\psi = E\,\psi,$](img2856.png) |
(1255) |
which only involves the radial coordinate
. It is easily demonstrated that
anti-commutes with
. Hence, given that
takes the form (1125), and that
, we can represent
as the matrix
![$\displaystyle \epsilon = \left(\begin{array}{rr}0&-{\rm i}\\ [0.5ex]{\rm i}&0\end{array}\right).$](img2858.png) |
(1256) |
Thus, writing
in the spinor form
![$\displaystyle \psi = \left(\begin{array}{c} \psi_a(r)\\ [0.5ex]\psi_b(r)\end{array}\right),$](img2859.png) |
(1257) |
and making use of (1222),
the energy eigenvalue problem for an electron in a central field reduces to the following two coupled radial differential equations:
Next: Fine Structure of Hydrogen
Up: Relativistic Electron Theory
Previous: Electron Spin
Richard Fitzpatrick
2013-04-08